Physics World em Agosto, 2014
The cutting-edge vacuum technology under development at Brazil’s new synchrotron, Sirius, will allow more stable and brighter X-ray beams for the study of matter at shorter length and timescales, describes Rafael Molena Seraphim
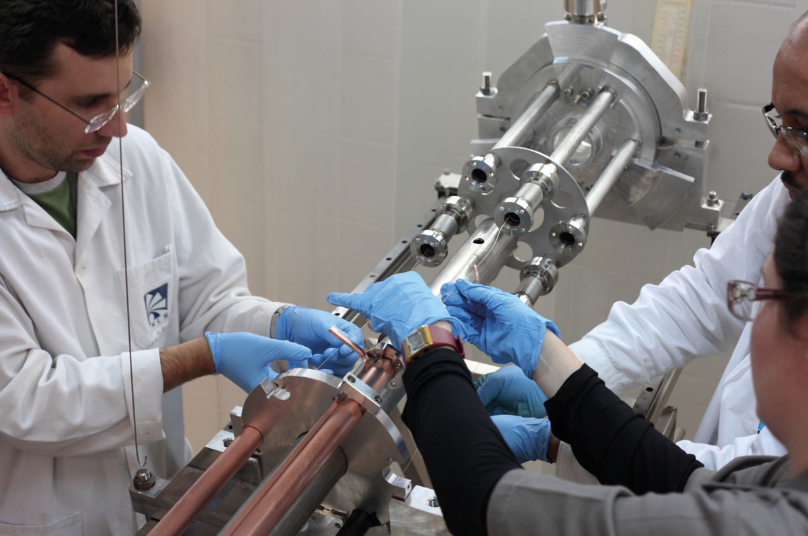
High production Intertwisted titanium, zirconium and vanadium wires being assembled into Sirius vacuum chambers in the NEG-coating rig at the Brazilian Light Source Laboratory. These are then inserted into a solenoid where a DC magnetron sputtering process completes the coating process. When fully loaded, the rig can simultaneously coat six 3.2 m-long vacuum chambers. (LNLS)
In the past few decades, synchrotron light sources have significantly advanced our knowledge of the structure and properties of materials. Based on electrons travelling around large storage rings, synchrotrons produce high-brilliance radiation ranging from the infrared to hard X-ray region of the spectrum. By tapping off this radiation and sending it along numerous beamlines that fan out tangentially from the storage ring, many different and unique experiments can be carried out simultaneously. In addition to advanced X-ray crystallography and imaging methods, synchrotrons allow scientists to link the atomic-scale structure of materials with their macroscopic properties and increasingly are able to characterize working devices such as solar cells in their native states.
Driving synchrotron science forward is the quest for ever-smaller beam “emittance” – a measure of the lateral spread of the electron beam. This results in a brighter and more collimated X-ray output, allowing the study of smaller features and faster processes. Around 50 synchrotrons of varying sizes and capabilities are in operation worldwide, and some of the top facilities are planning upgrades based on storage rings with very low emittance. The Advanced Photon Source (APS) in the US, the European Synchrotron Radiation Facility (ESRF) in France, and SPring-8 in Japan are all proposing ambitious upgrades, while other labs are building entirely new machines – namely MAX IV in Sweden and Sirius in Brazil.
Sirius will replace Brazil’s existing, second-generation synchrotron with one of the brightest X-ray sources on the planet. Boasting a very low emittance of 0.28 nm rad, it will also be the first third-generation light source in Latin America. Ground works for the new facility are complete and construction is scheduled to start at the end of this year, with engineering teams already deeply engaged in the design, prototyping and R&D of the machine’s subsystems. Commissioning is planned to start in mid-2016 and the first beam for users in mid-2017, which is a tight schedule.
Distributed environment
In all synchrotron light sources, an ultrahigh-vacuum environment is essential in order to accelerate electrons and extract the radiation that they produce. The most demanding component of such a facility is the storage ring, which must operate at pressures bellow 10–9 mbar to minimize beam-gas scattering and other effects that reduce the lifetime of the electron beam. Since the cross section of the vacuum chamber is very small compared with its length (the Sirius storage ring will have a circumference of 518 m and a chamber diameter of 24 mm, for instance), these machines tend to be pumped in a discrete or distributed manner.
Most modern storage rings use discrete pumping, whereby hundreds of pumps are installed at intervals of around 1.5 m or less, which requires vacuum chambers with good conductance and sufficient longitudinal space in which to place the pumps. But this approach goes completely against the design of next-generation synchrotrons, which in order to reduce beam emittance will guide electrons more gradually around the ring. Technically, Sirius is based on a “multi-bend achromat” design that involves many small-aperture magnets and leaves little space for other components. In total, the storage ring will comprise 20 “five-bend cells” and 20 straight sections.
Distributed pumping based on non-evaporable getter (NEG) technology offers a more effective option for such a compact “lattice”. NEG coatings, which were developed by the CERN particle-physics lab, are based on thin films that coat the inner surfaces of a vacuum chamber and have a chemical affinity for gas molecules. CERN has used the technology extensively in the beam pipes of the Large Hadron Collider, which requires extreme vacuum conditions. Today, NEG coatings are a proven industrialized technology used in many other settings. The first synchrotron to extensively use NEG coatings was France’s national light source Soleil, with 56% of the machine exploiting the technology. Sirius and MAX IV will be the first synchrotron light sources to base vacuum pumping of the storage ring mainly on NEG coatings, with more than 95% of the vacuum chambers being coated.
The Sirius challenge
The highly compact lattice of the Sirius storage ring leaves very little space for components and therefore requires narrow vacuum chambers, which are harder to pump. Another challenge is that the vacuum chambers and components must provide a continuous electrical path to minimize the impedance of the machine, which can affect beam stability by introducing electromagnetic wake fields. The most common materials used in third-generation light sources are stainless steel and aluminium, but we have chosen to build the Sirius vacuum chamber from oxygen-free silver bearing (OFS) copper. The higher electrical conductivity of this material minimizes the machine’s impedance, while its improved thermal conductivity makes it better at absorbing unused synchrotron radiation. OFS copper also has a higher annealing temperature, which is convenient for NEG coatings because vacuum chambers need to be heated to a temperature of at least 200 °C to activate the coating. Most of the Sirius storage ring vacuum chambers will have a circular cross section with an inner diameter of 24 mm and a wall thickness of 1 mm.
Since the chambers also have to absorb unused synchrotron radiation, narrow copper cooling pipes will be attached to their outer side. But the machine’s compact lattice means that other sections of the storage ring – especially where the X-rays are siphoned off and sent down various beamlines towards experimental targets – demand more complex chambers. This makes the NEG coating extremely challenging. Sirius will have around 450 chambers in total, and even the circular cross-section chambers inside the multipole and bending magnets (where there is no need to extract radiation for the beamlines) do not have a simple manufacturing process.
Three joining processes will be needed to manufacture every single chamber to ensure that the copper does not become annealed and distorted. Vacuum brazing will be used to join short copper adapters to the stainless-steel vacuum flanges, while tungsten inert gas (TIG) welding will be used to weld these components to the copper vacuum chambers. A robotized TIG welding station gives us precise control and is suitable for complex geometries, but neither TIG welding nor vacuum brazing are suitable for attaching the copper cooling pipes to the vacuum chambers because of the potential for distortion or annealing, respectively. Here, vacuum soldering at a temperature of about 330 °C is being developed to produce joints for thermal contact.
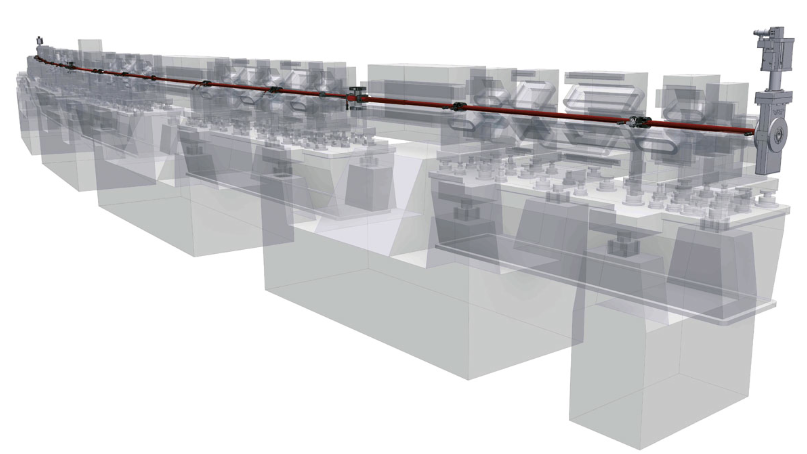
Fine thread The Sirius vacuum chamber, inside which electrons will circulate while emitting synchrotron radiation, has to weave its way through a highly compact arrangement of magnets and other components (grey) and needs complex chamber shapes and more than 450 individual sections. (LNLS)
NEG coatings
Since all the Sirius vacuum chambers will have small vacuum conductance, on account of their narrow cross section, NEG coatings are paramount. The host lab of Sirius, the Brazilian Light Source Laboratory (LNLS), has signed a licence agreement with CERN to use the coating technology and also has its own NEG coating facility to produce the Sirius vacuum chambers. Built last year by the LNLS engineering teams at a cost of around R$300,000 (about £80,000), this is one of the few places in the world where NEG coatings can be produced according to standards determined by CERN – offering the ability to coat vacuum chambers up to 3.2 m long and 450 mm in diameter (see top image).
To ensure that the NEG coatings have good adhesion and pumping properties, the surfaces of the vacuum chambers must be completely free of contaminants. We therefore developed a special cleaning process based on a recirculation system where only the inner surfaces of the vacuum chambers are exposed to the etching solutions, which also reduces workers’ exposure to harmful substances. Although NEG coatings for the simple circular vacuum chambers are currently in their final design stages, the coating procedure for the complex vacuum chambers is still under development.
Following the NEG coating process, the vacuum chambers will be filled with nitrogen and stored in batches according to their assembly in the storage ring. Since the coatings must be activated by heating them in situ (in a procedure called a bake-out), Sirius requires lots of bellows to accommodate the chamber’s expansion and this can lead to a higher machine impedance. Also, the heating tapes wrapped around the chambers to heat them up must be very thin for those chambers inside the multipole and dipole magnets. For this reason, we have developed a customizable thin polyimide heating tape in conjunction with Brazilian company EXA-M Instrumentação do Nordeste, which is one of the first successful examples of LNLS’s partnership with Brazilian companies to build Sirius.
Global ambition
Further Brazilian firms are being encouraged to take part in Sirius, and collaborations between LNLS and other laboratories are in progress. One involves CERN, where there is a mutual interest between our vacuum teams in studying the behaviour of surfaces exposed to synchrotron radiation. These studies will allow a better understanding of the Sirius vacuum chambers as well as an opportunity for CERN to better understand the proposed new surface technologies for the LHC chambers.
Sirius will soon be one of the world’s brightest synchrotrons, opening new frontiers for research across materials science and also serving as a stepping stone to a diffraction-limited storage ring – a so-called ultimate synchrotron. The design is pushing synchrotron technology to the limit, especially concerning the different and unique concepts proposed for the vacuum system. All of the vacuum chambers and components must be designed and manufactured according to tight requirements, not just in terms of vacuum-system specifications but also to maximize the scientific performance of Sirius.
There are still many challenges to overcome with the Sirius vacuum system, such as the manufacture and NEG-coating of the complex-shape chambers. But once operational, Sirius will provide research from Brazil and the rest of Latin America with the opportunity to develop cutting-edge science in many fields and put nations from this region on the scientific map.